

Whether transportation networks are assessed on the microscopic or the macroscopic scale, and irrespective of the imaging technique, the first step is the throughput automated image analysis, involving noise reductions, filtering, and thresholding, followed by morphological processing to achieve a firm skeletonization. In recent studies it has been shown that investigating these circulatory systems can benefit largely when assessed from a network-based perspective, not only through quantification and understanding of transport processes in hierarchical and loopy networks, but also through reconstruction of vascular architectures from imperfect data. There is immense interest in identifying how the branching network architecture, resource distribution, flow, and damage resilience affect biological function and how do these features relate to the allometric scaling theory. Many biological fluid transport networks, such as branching patterns of blood vessels, venation in plant leaves, fungal networks, and slime molds are optimized to redirect flow as dictated by the needs of the system. It can potentially offer insights for other reversible motions observed in plants induced by differential turgor and water transport through the xylem vessels, as well as engineering applications. This flow-controlled mechanism of shape transformation was inspired by the blooming of flowers through the unfolding of petals. To quantify these effects, we explore the correlation between local Gaussian curvature and relative stored fluid content in each hierarchy by using linear regression, which reveals that stronger correlations could be induced by less densely connected major veins. We investigate the effects of various designs of the flow network, including overall hydraulic traits (resistance and capacitance) and hierarchical architecture (arrangement of major and minor veins), on the specific dynamics of membrane shape transformation. The shape of the surface is modeled by a spring network produced by a tethered mesh discretization, in which local bond rest lengths are adjusted instantaneously according to associated local fluid content in the capacitors in a quasi-static way. We simulate the time-dependent fluid distribution in the flow network based on a spatially explicit resistor network model with local fluid-storage capacitance. In order to computationally study the hydraulically induced differential swelling and buckling of such a membrane, we develop a network model that describes both the membrane shape and fluid movement, coupling mechanics with hydrodynamics. Different parts can hydrate and swell unevenly, prompting a differential expansion of the surface.
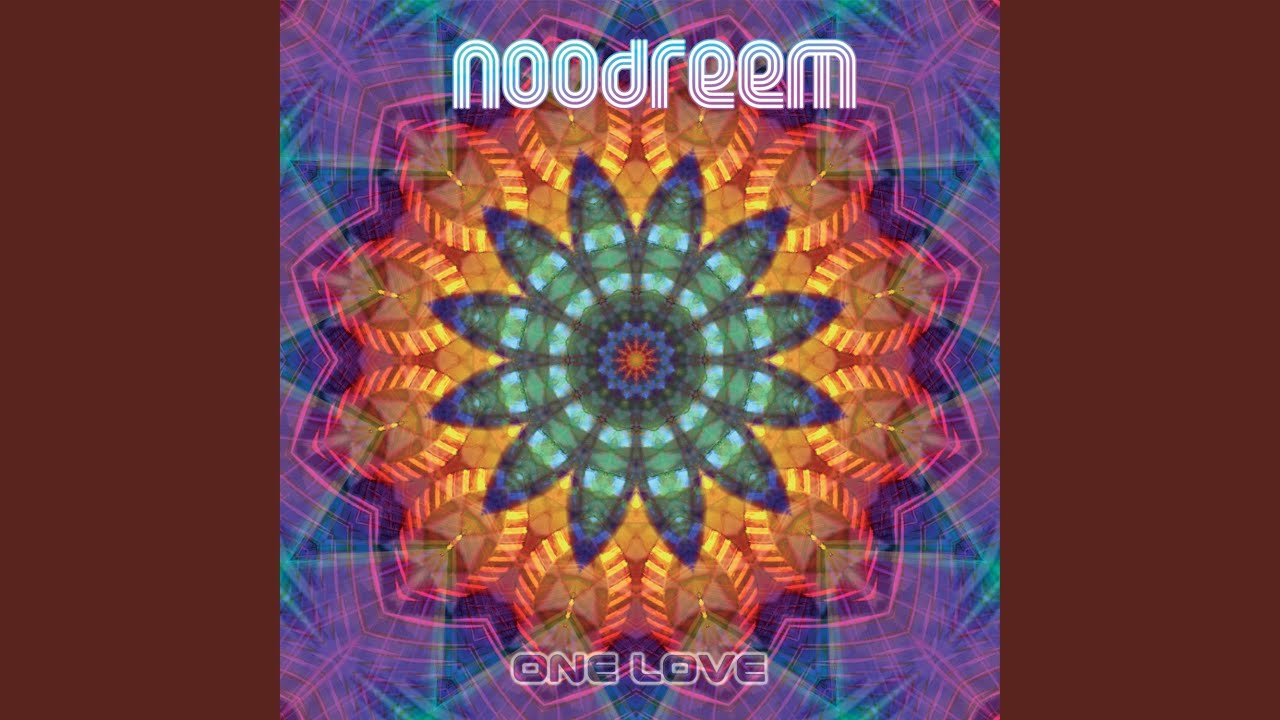
Fluid absorption and storage eventually cause the material to locally swell.

The mechanical properties of a thin, planar material, perfused by an embedded flow network, can be changed locally and globally by the fluid transport and storage, resulting in small or large-scale deformation, such as out-of-plane buckling. Our model also predicts a quarter-power scaling relationship between conduction time delay and body size. Notably, our findings reveal that the branching of axons and peripheral nervous system neurons is mainly determined by time minimization, while dendritic branching is determined by power minimization. We test our predictions for radius scale factors against those extracted from neuronal images, measured for species that range from insects to whales, including data from light and electron microscopy studies. Here, by constructing biophysical theory and testing against empirical measures of branching structure, we develop a general model that establishes a correspondence between neuron structure and function as mediated by principles such as time or power minimization for information processing as well as spatial constraints for forming connections. Classifying neurons according to differences in structure or function is a fundamental part of neuroscience. Neurons are connected by complex branching processes-axons and dendrites-that process information for organisms to respond to their environment.
